How UT Students are Fueling Energy Research
From advancing sustainability to enhancing efficiencies, student researchers are a part of UT’s energy story.
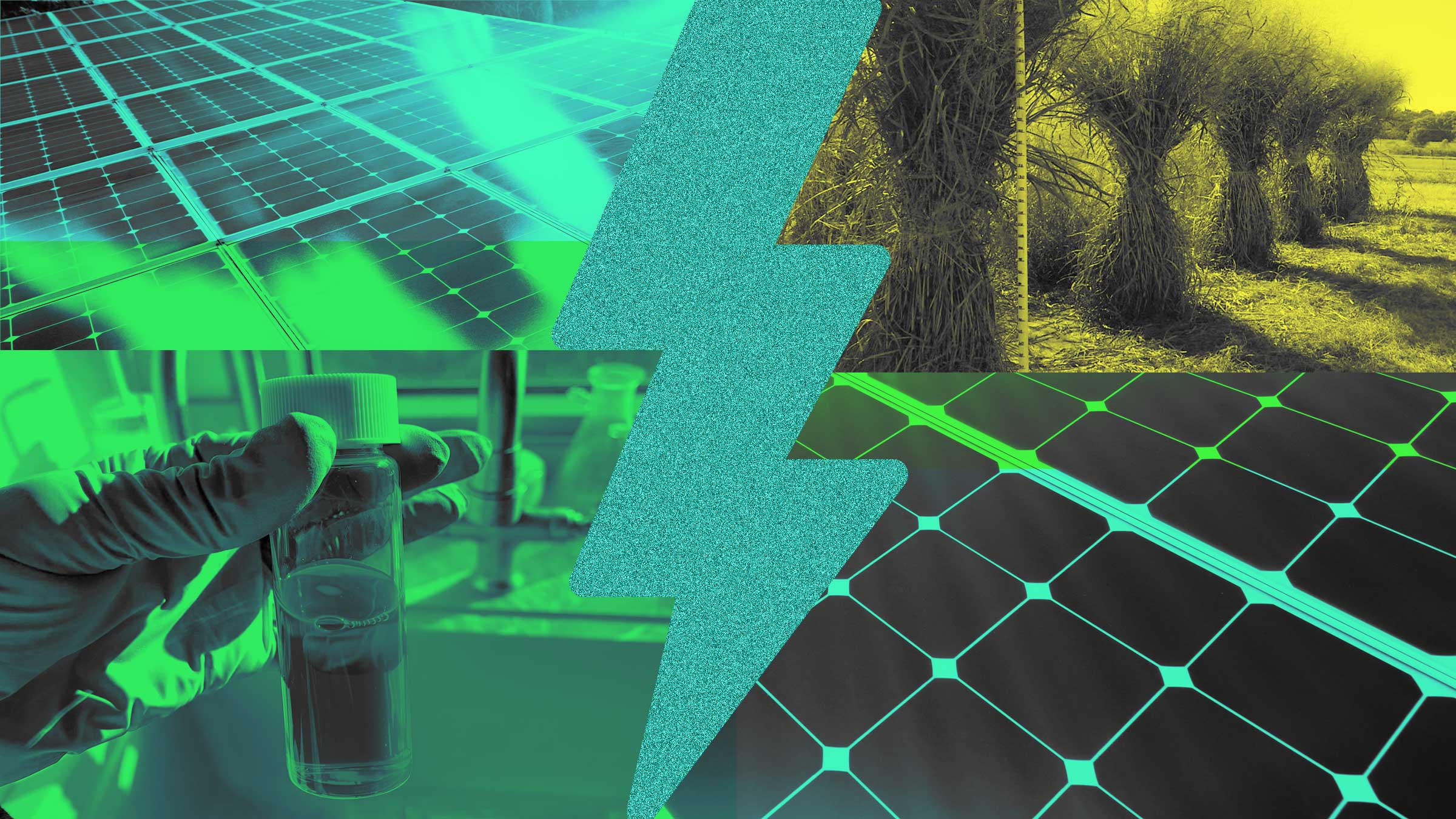
Photo-Illustration: Martha Morales
As UT Austin celebrates Energy Week, a gathering of energy leaders and researchers, it is worth remembering that the work on innovative energy research happens year-round. That’s in part because curiosity-driven undergraduate and graduate students at UT Austin, including in the College of Natural Sciences, are pursuing exciting new breakthroughs related to energy.
Optimizing solar panels
While solar panels are revolutionizing clean energy, solar cells have a limit to their efficiency due to inherent energy losses when absorbing high-energy photons. Tanner Volek, a fourth-year chemistry Ph.D. student who works in the lab of Professor Sean Roberts, is studying a process called singlet fission, which could improve solar cell efficiencies by decreasing the amount of energy lost as heat and doubling the number of electrons collected by the solar cell per photon absorbed.
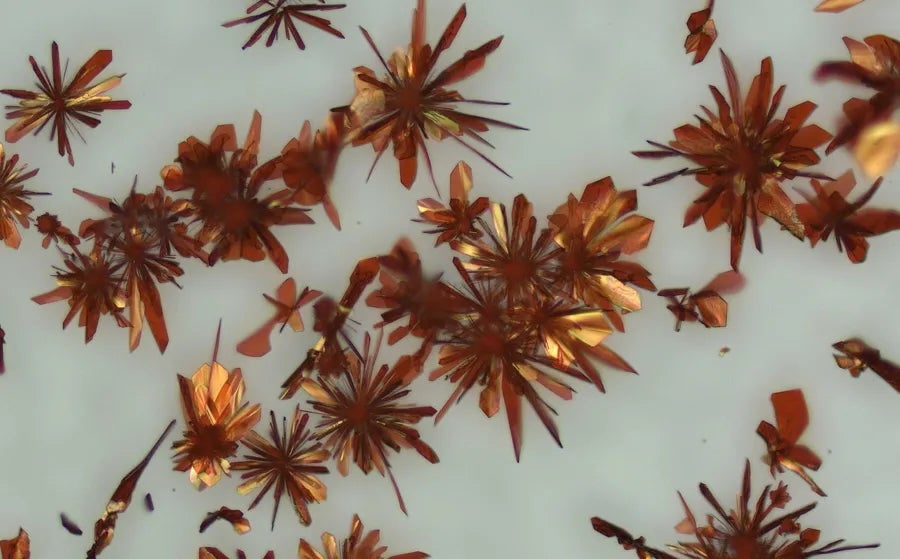
Molecular crystals of Diketopyrrolopyrrole, an organic chromophore that absorbs visible wavelengths of light, were grown via a physical vapor deposition method. Image by Tanner Volek.
“Normally, a solar cell absorbs a photon of light, and you get one excited electron,” Volek said. “But if we can utilize singlet fission, then we can take one photon and excite two electrons, so we can double the number of electrons that a solar cell can collect from a photon of light.”
In singlet fission, high energy photons are absorbed and converted into a lower energy state as one excited molecule “shares” its energy with neighboring molecule. In the future, solar panels could include materials capable of singlet fission to boost their efficiency and longevity.
Volek grows crystals out of organic chromophores, molecules that absorb visible light.
“I study processes like singlet fission in these crystals, and I relate it to their structure to try to learn something fundamental about how they convert one form of energy to another,” he said.
Volek said solar farms currently require vast amounts of space, but theoretical modeling has shown that singlet fission can improve a solar cell’s efficiency by around 30%.
“If we could make the solar cell 30% more efficient, that’s 30% of that space that we can save and move the solar cell farm closer to people who need it,” Volek said. “Making solar cells more efficient in general will make them more economically viable as a renewable resource.”
Sowing the seeds of sustainability
Andrew Eastland, a biology senior, is interested in the power of biofuels, or renewable energy that comes from living matter.
“Burning fossil fuels introduces carbon to the atmosphere that we have no way of putting back into the ground when we’re done,” Eastland said. “Plants, because they get their carbon from the atmosphere, have this great cyclical exchange where we can run net neutral, while still meeting our energy demands.”
Switchgrass has potential to be a powerful biofuel producer as it has more cellulose per unit of mass than corn, which could allow companies to get more ethanol, a key biofuel, out of switchgrass than from a corn plant of a similar size. Eastland works with Thomas Juenger, a professor of integrative biology, to investigate which genes help Hall’s panicgrass, a sister species to switchgrass, survive when the plants are exposed to stressful situations, such as environments with drought or toxic metals. To do this, Eastland takes mutated varieties of the grasses and analyzes where those mutants struggle and where they thrive.
“We’re hoping it can give us an idea of where we could plant switchgrass if we wanted to start getting biofuel on a larger scale,” Eastland said. “There’s a lot of land in the U.S. that is polluted with factory waste, and we think that switchgrass has the potential to survive in those environments. But we have to figure out whether it’s genetically capable.”
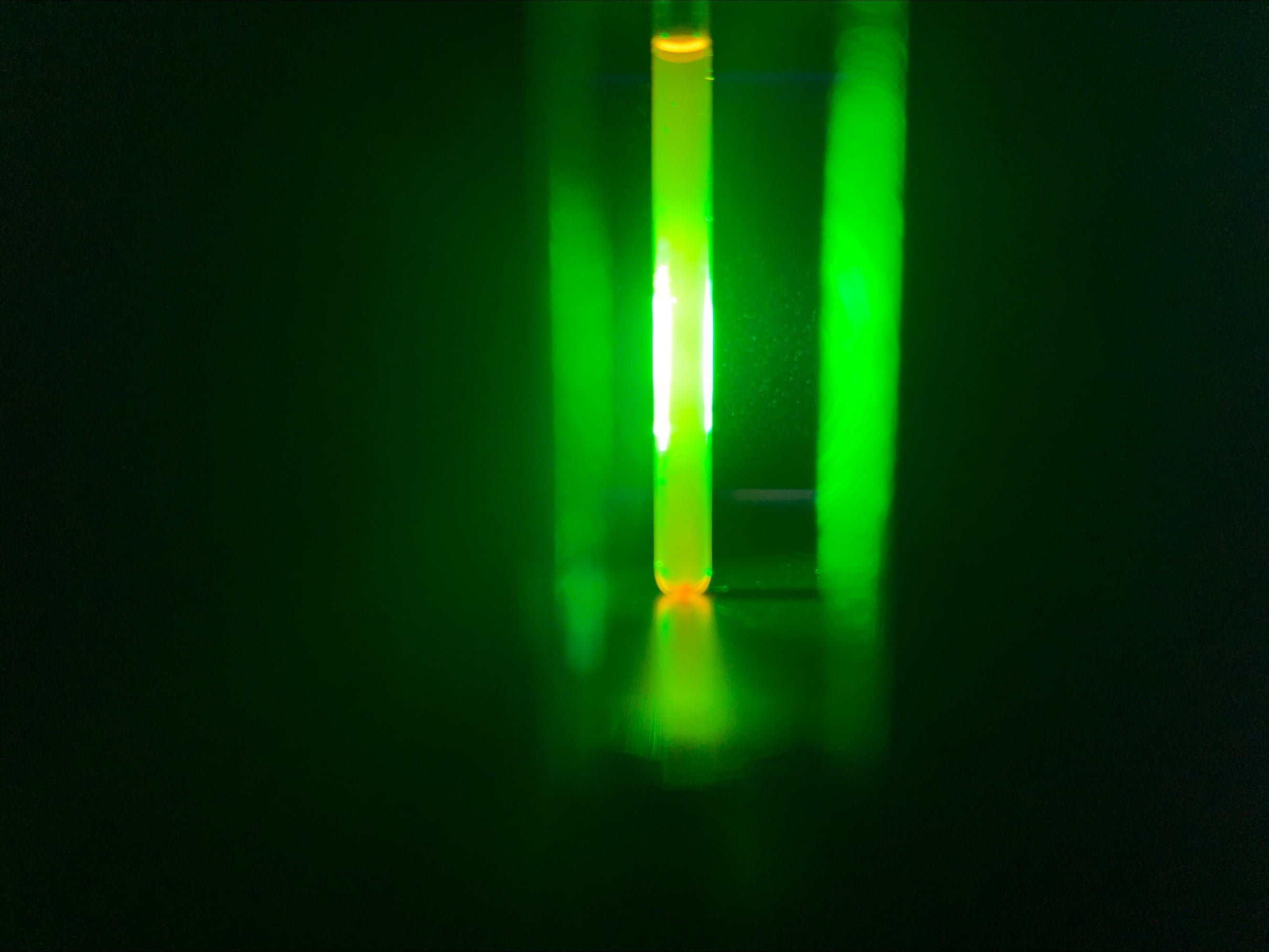
Vial of BODIPY being analyzed using electron paramagnetic resonance (EPR) spectroscopy while being illuminated with green light. Photo by Madison Handojo.
Transforming 3D printing
Precise 3D printing today, unfortunately, is both energy-intensive and requires costly ultra-violet (UV) light, which is dangerous to living cells. Madison Handojo, a junior chemistry major, works with Zak Page, an assistant professor of chemistry, to study photocatalysts called boron-dipyrromethene (BODIPY) dyes. These dyes, used as additives in 3D printing, are pigmented substances that absorb light at a specific wavelength, and they could help open a path to a more energy-efficient alternative to what is required for certain types of 3D printing today.
Handojo started her research journey as a student in the Materials in Color Freshman Research Initiative stream, which Zak Page serves as the principal investigator for. The summer after her freshman year, she began research with the Page Lab.
“It was very fun because I had my first experience with synthesis and 3D printing there,” she said. “I developed a near-infrared absorbing photocatalyst, which absorbed a very weak energy of light to use in polymerizations.”
Handojo said she especially enjoys considering how her research fits in at an industrial scale.
“If you can use a longer and lower energy wavelength of light to optimize a polymer system, you should try and do that,” Handojo said, “because that small amount of energy you’re using unnecessarily can lead to big costs.”