Peering Inside a Quantum Computer Creates New Phases of Information
Physicist Matteo Ippoliti helped explore how measurements can alter information states in an innovation created by Google.
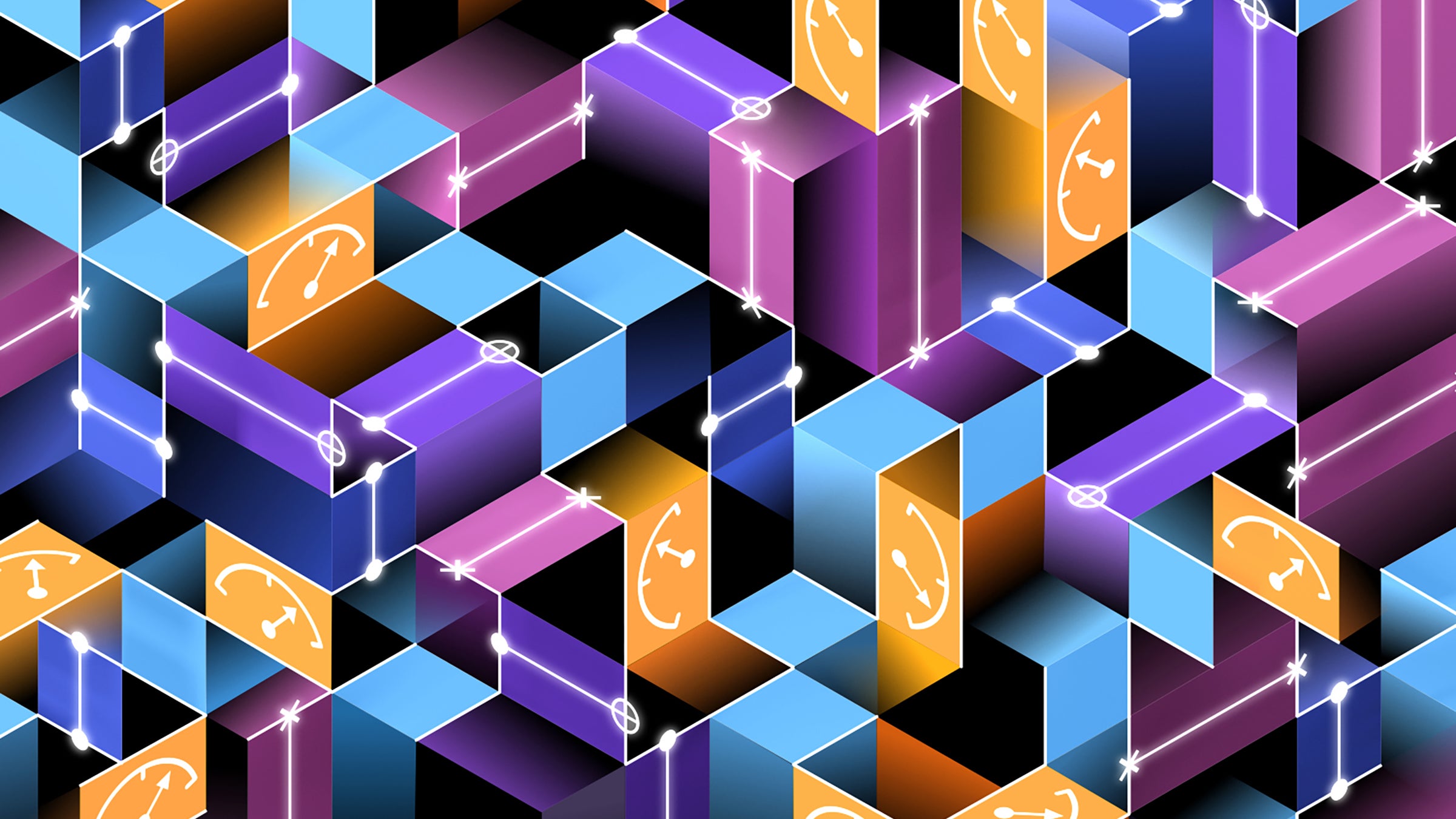
Illustration credit: Google Quantum AI, designed by Sayo-Art.
A quirk of quantum physics is that when no one’s looking, a particle behaves in weird ways, like existing in multiple places at the same time or sharing an intimate bond with another particle far, far away. But take a measurement, and suddenly, that weirdness evaporates and the particle behaves in a boring, classical way. A burning question for physicists is how that transition from the quantum to the classical world happens.
Matteo Ippoliti, an assistant professor of physics at The University of Texas at Austin, and his colleagues report in the journal Nature on their explorations of how measurements affect information in Google’s most powerful quantum computer, built around a 70-qubit chip called Sycamore.
The team, led by Xiao Mi and Pedram Roushan at Google Research and Vedika Khemani at Stanford University, demonstrated that taking certain kinds of measurements on a quantum computer gives rise to different structures, or phases, of quantum information.
“This is notable because measurement is usually just a spectator, while here it is really an actor that plays a fundamental role,” said Ippoliti, who worked on the theoretical aspects of the project as a postdoctoral researcher at Stanford before joining the faculty at UT Austin this year.
This is by far the largest system in which such a quantum information phase transition has been observed. This required sophisticated new tools and methods.
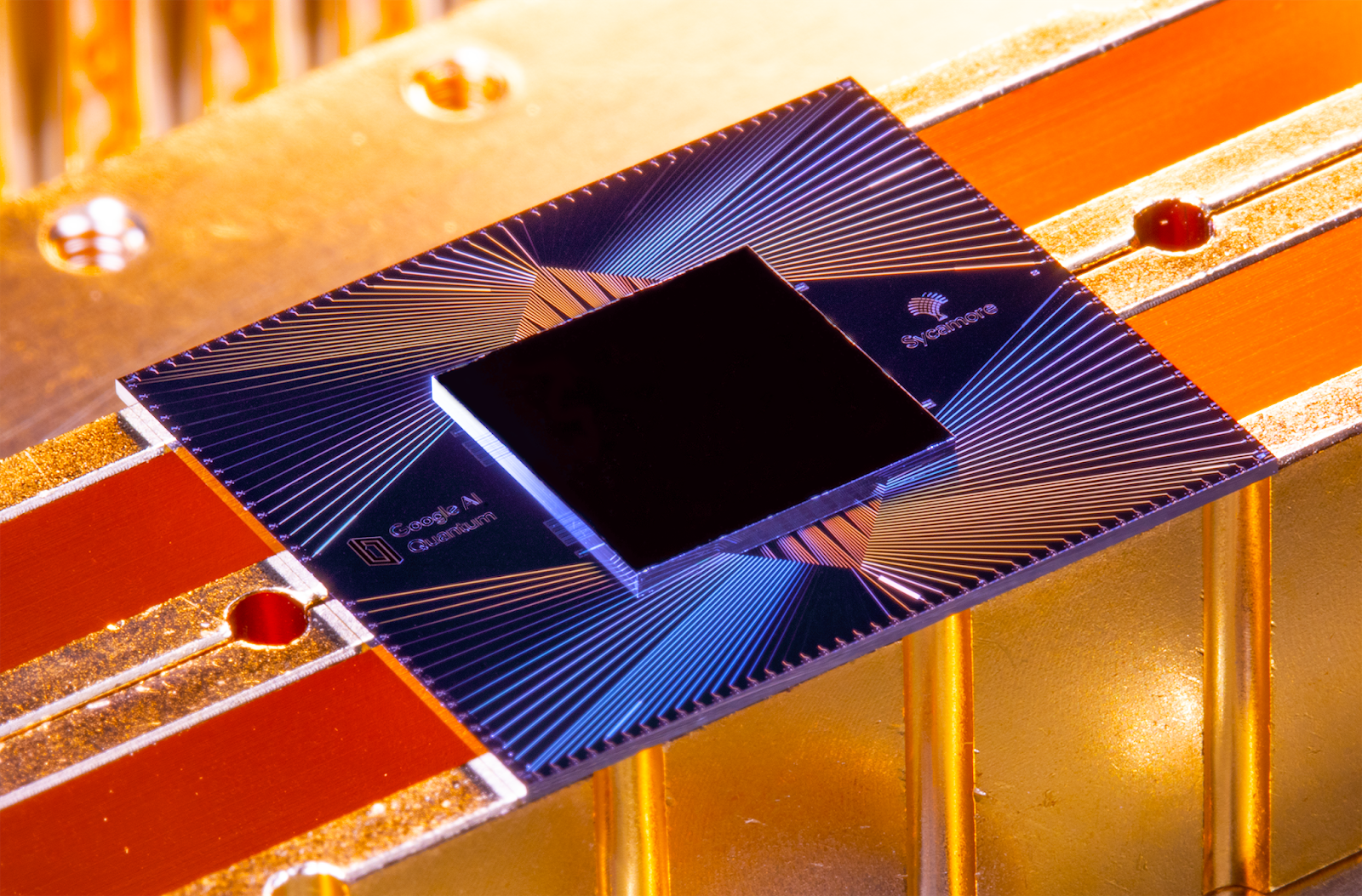
Photograph of Google’s Sycamore processor. Credit: Erik Lucero, Research Scientist and Lead Production Quantum Hardware.
When the team took measurements and induced one particular phase of information, they observed quantum teleportation, a phenomenon where quantum states are sent from one point in space to another instantaneously. That might seem like a powerful new tool for sending information faster than the usual speed limit in electronics, namely light speed. But there is a big catch: information sent this way can’t be controlled – it appears as purely random on the receiving end. So there doesn’t seem to be a way to practically harness this effect for computing.
Still, there may be more immediate, practical applications of their work; for example, in managing noise in future, more powerful quantum computers.
Quantum computers—at least the current generation—are extremely susceptible to what’s referred to as noise: tiny tickles from the surrounding environment, such as cell phone signals, changes in Earth’s magnetic field, and even cosmic rays, that can lead to errors in the computation. As engineers and physicists build ever larger quantum computers, they need tools for quantifying noise and mitigating it.
“From the signal we measured we can learn about noise in the system, that is to say, imperfections in the quantum computation that cause it to lose accuracy over time,” said Ippoliti. “This is very important for near-term quantum computers, where we don’t yet have the ability to correct errors.”
When the paper has been published online, it will be available at the following URL: https://www.nature.com/articles/s41586-023-06505-7