UT Scientists Spied a Skyrmion. What is That?
This, and six other questions about a recent first in physics, answered.
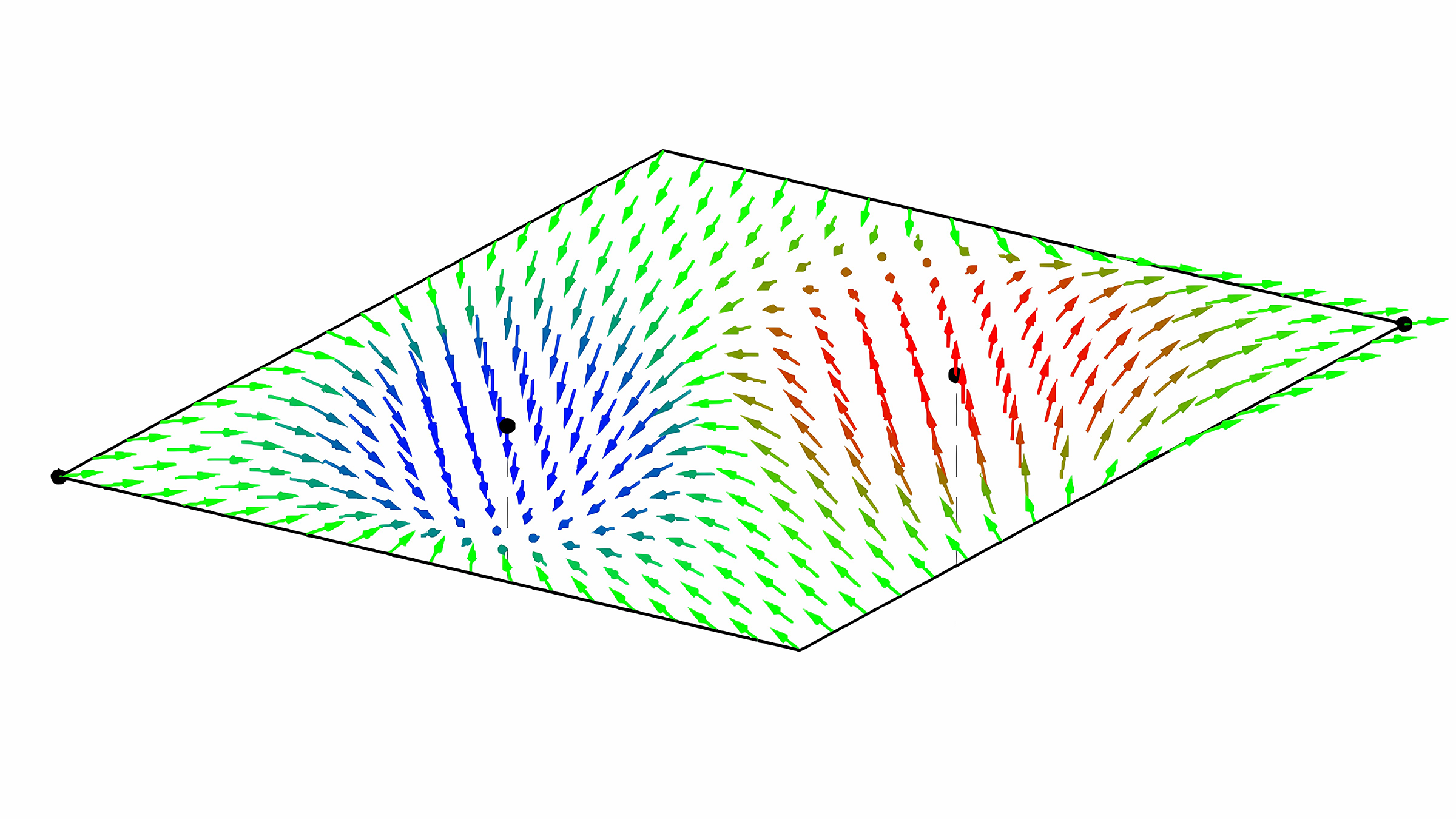
A UT-led team observed an interlayer skyrmion—a swirling pattern of electric dipoles in an ultrathin material—for the first time in tungsten selenide (WSe2). Arrows indicate the direction in which electric dipoles point, with blue pointing down and red pointing up. Illustration credit: Fan Zhang/University of Texas at Austin.
A University of Texas at Austin-led team of researchers has observed for the first time an exotic structure called an interlayer skyrmion—something that had been theorized but never before demonstrated experimentally. Interlayer skyrmions hold promise for new materials that could enable next-generation technologies, like improved quantum computers, the scientists say.
Funding support was provided by the National Science Foundation, the Department of Energy, the U.S. Air Force and the Welch Foundation.
What in the world even is an interlayer skyrmion?
An interlayer skyrmion is a swirling pattern or texture in a highly specialized thin material. Scientists have suggested that this kind of structure could someday be useful, for example, by quantum computers to make them more impervious to noise. The skyrmion’s pattern is made up by the directions of its electric dipoles, which are tiny pairs of electric charges in the material with a negative charge on one end and a positive charge on the other. In the accompanying illustration, each arrow represents the direction of an electric dipole. They can point in any direction—in some places, they point down (blue), in others, they point up (red). The overall pattern of these ups, downs and sideways swirls is an interlayer skyrmion.
Why are we talking about this now?
As reported in Nature Physics, Chih-Kang Shih, Allan MacDonald and fellow UT physicists managed to produce the skyrmion by stacking two atomically thin sheets of tungsten selenide (WSe2) on top of each other and rotating the sheets relative to each other by a small angle. The result is known as a twisted bilayer material. Using a scanning tunneling spectroscope, they were surprised to discover the exotic structure described above, which had been theorized for a similar material, but never observed in WSe2. Technically, the observed object is an “interlayer pseudo-spin skyrmion.”
What are the scientists saying about it?
“The general public may be most interested in potential quantum computer applications, but I’m most excited to observe for the first time a structure that has only been theoretically predicted before,” said Shih, a physics professor who co-led the research with MacDonald, who is also a professor, and postdoctoral researchers Fan Zhang and Nicolas Morales-Duran (now at Flatiron Institute).
What real-world applications could follow from the discovery?
One of the biggest challenges to building quantum computers is that noise in the surrounding environment—such as cell phone signals, changes in Earth’s magnetic field and even cosmic rays—can cause errors in components that store and process information, called qubits. Physicists have suggested a possible solution: instead of using conventional qubits made of individual ions, photons or atoms, build them with so-called “topological qubits” that store and process information in the topological properties of a physical system.
Why is an interlayer skyrmion particularly important for the noise problem?
What makes interlayer skyrmions promising for quantum computing is that when electrons pass through the material, the skyrmion imparts a special property to the electrons that physicists call a “non-trivial geometric phase.” A key takeaway from this larger, complicated mathematical concept is this: electrons that are stamped with this property tend to retain it, even when buffeted by noise in the surrounding environment. This resilience makes certain skyrmions strong candidates for building robust topological qubits and overcoming the noise problem in quantum computers.
So what comes next?
Shih cautioned that it may be a long time before working topological qubits are built using materials exhibiting interlayer skyrmions. Researchers have tried this approach for years without definitively building one.
“There are several schemes that have been proposed for building a topological qubit,” Shih said. “For example, Microsoft’s Majorana 1 based on an indium arsenide-aluminum wire platform. So far, it’s unclear which one will be most promising.”
“Our paper offers an alternative candidate material for topological qubits,” Shih said. “We still don’t know which one will win.”
The other UT Austin co-authors are: Yanxing Li, Xiaohui Liu, Fu-Xiang Rikudo Chen, Hyunsue Kim and Xiaoqin “Elaine” Li. Other co-authors are: Wang Yao at The University of Hong Kong; Jung-Jung Su at National Yang Ming Chiao Tung University (Hsinchu, Taiwan); Yu-Chuan Lin, Chengye Dong and Joshua A. Robinson at Pennsylvania State University; and Kenji Watanabe and Takashi Taniguchi at the National Institute for Materials Science (Tsukuba, Japan).