Can General Relativity, at 100, Withstand Some Holes?
Answering some of the biggest questions in astrophysics—for example, about black holes and the origin of the universe—might require overhauling general relativity.
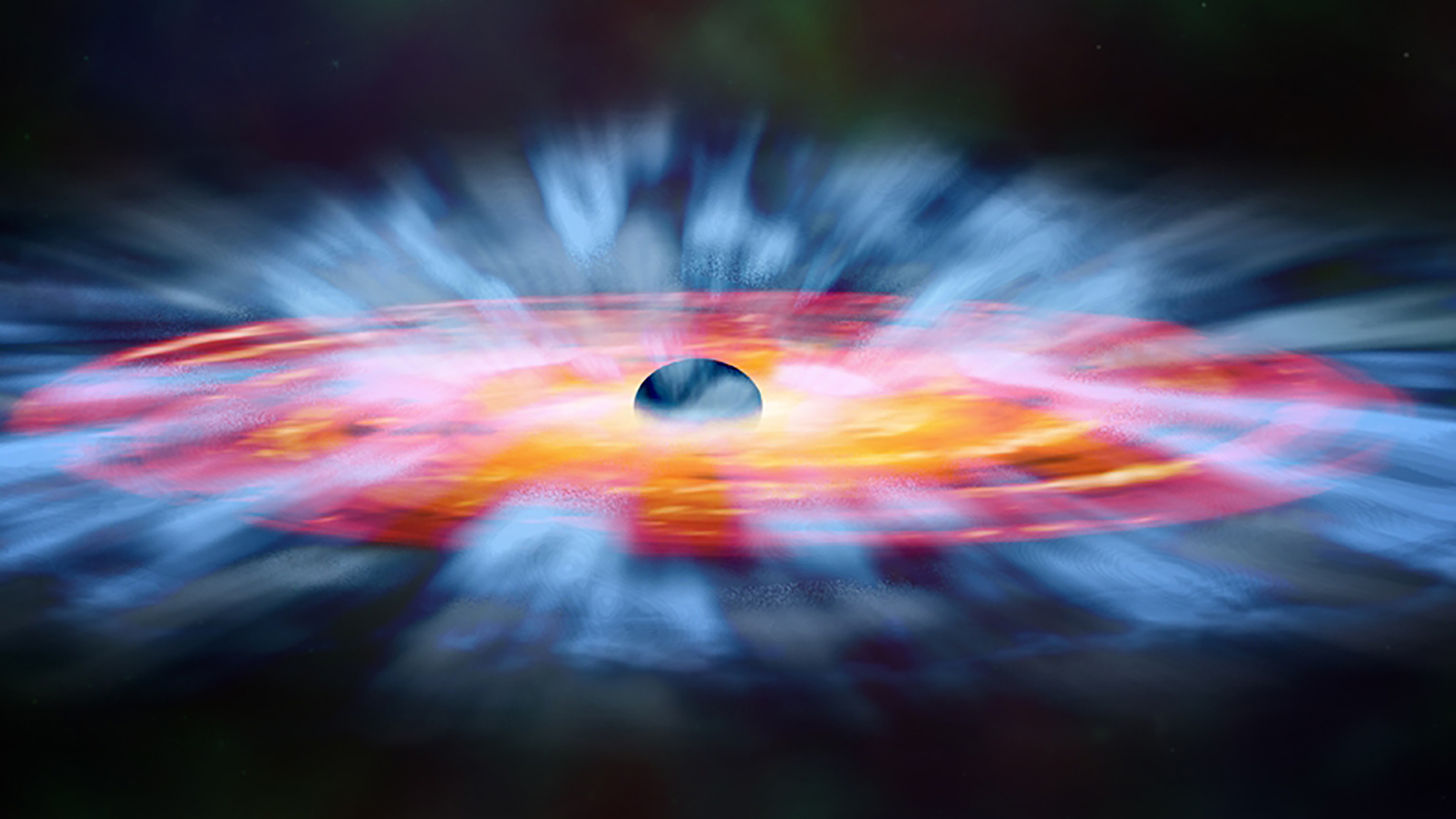
In this artist’s illustration, turbulent winds of gas swirl around a black hole. Some of the gas is spiralling inward towards the black hole, while some is blown away. Credit: NASA and M. Weiss (Chandra X-ray Center)
This is the first of a three-part series on general relativity.
In November 1915, Albert Einstein stood before his colleagues in the Prussian Academy of Sciences and unveiled a set of equations that would forever change the way we see the universe. The Theory of General Relativity, Einstein's description of gravity, explained the motions of everything we see in the universe.
One of Einstein's key insights is that matter warps space and time, much like a bowling ball causes a trampoline to sag. That's why when light from a distant star passes near a massive object like our sun, its path is bent slightly, and why a clock on Earth ticks slower than a clock in an orbiting satellite. (If engineers hadn't taken that into account, the GPS system in your car wouldn't work.)
So far, general relativity has held up well to various tests scientists have thrown at it. But there are signs that it's incomplete.
Physicists say that within black holes and in other areas of extreme mass or energy density, the mathematical equations that explain so much of the universe blow up. And in some cases, relativity clashes with another great pillar of physics: quantum mechanics, which describes subatomic particles and how they interact.
Scientists now are drawn to a question: what happens in the places where theories collide?
"In 100 years, general relativity has never been shown to be wrong," says Willy Fischler, a professor of physics. "Neither has quantum mechanics. But when we put the two together, we have problems."
As scientists at The University of Texas at Austin work to answer some of the biggest questions in astrophysics—for example, about black holes and the origin of the universe—some say finding the answers might require overhauling general relativity.
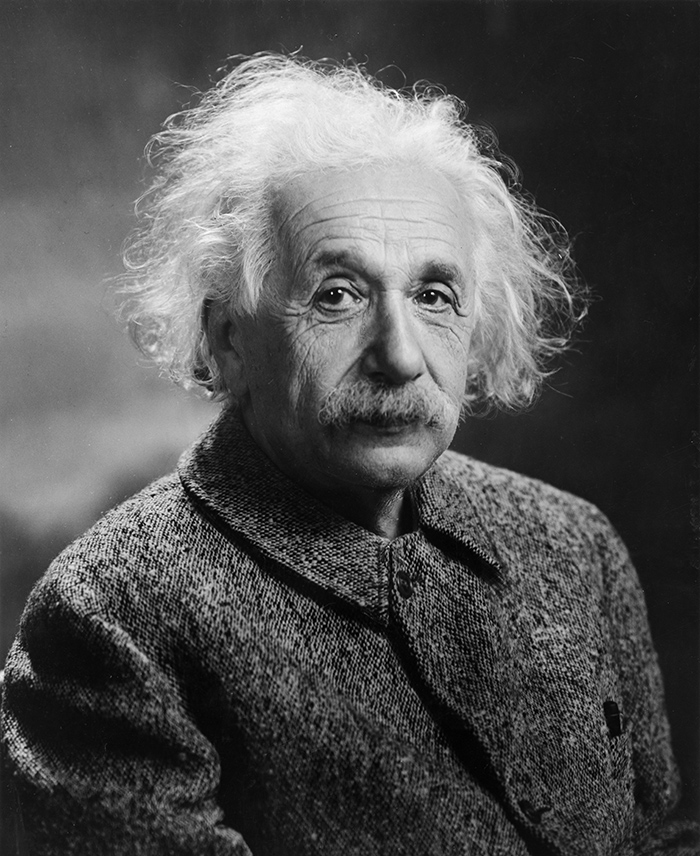
Albert Einstein 1947
Inside Black Holes
When a very large star runs out of nuclear fuel and breathes its dying breath, it collapses into a black hole, an object so dense that not even light can escape it. A star ten times as massive as our sun would squeeze down into a ball about the width of Austin.
From a distance, general relativity does a great job describing black holes. But take a closer look and things get weird.
First, consider what happens at the very heart of a black hole. According to general relativity, mass and energy become infinitely dense and space and time become infinitely curved. As such a point, called a singularity, the steps involved in solving Einstein's equations become infinite. Physicists run into the same head-scratching conundrum when they try to study another singularity—the birth of our universe as a hot, dense plasma in the Big Bang.
Second, consider what would happen if you fell into a black hole. According to general relativity, you would first cross something called the event horizon, the outer edge of the black hole, beyond which not even light can escape. Then as you continued falling, the black hole would shred you apart, atom from atom, like some cosmic garbage disposal apparently deleting information about you forever.
Meanwhile, an observer outside of the black hole would see you approach the black hole but never actually cross over the event horizon. With some as-yet-uncreated technology, the observer would detect a slight increase in the mass and rotation of the black hole.
Yet according to quantum mechanics, information can never be truly lost. This is called the information paradox.
"We have problems in the context of black holes. This clash means there's something missing in our understanding, and we have the chance to make a big leap in understanding, to change paradigms," Fischler says. "Something has to give here. What is it in the description of either one or both of these theories that has to be amended?"
Part of the answer might be that, when an object crosses the event horizon, it leaves behind a two-dimensional imprint on the surface of the horizon with all the information about its state, similar to a hologram. So from the point of view of quantum mechanics, the object could fall in, but information about it would not be lost.
Ultimately, to resolve the information paradox and make sense of what happens in a singularity, physicists might also need to develop a theory that reconciles general relativity and quantum mechanics. Steven Weinberg, a Nobel laureate and professor at UT Austin, and others have long sought a theory of everything that would do just that.
"String theory is probably the most promising way to unify the two theories," says Weinberg.
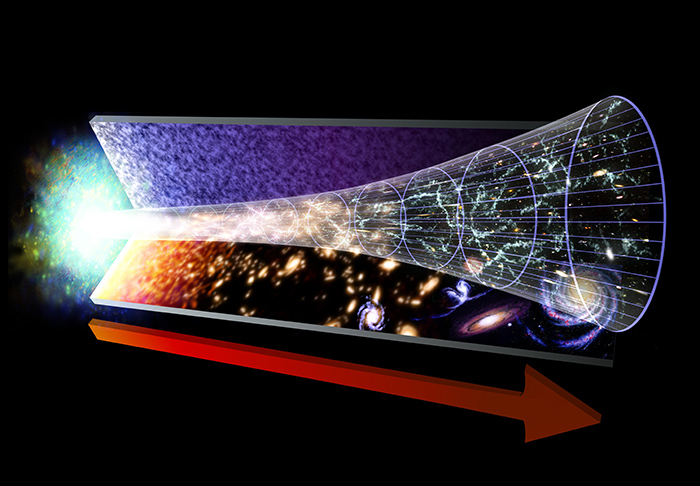
The evolution of the Universe, starting with the Big Bang. The red arrow marks the flow of time. Credit: Dana Berry (Skyworks Digital)/NASA
How Did our Universe Start?
String theory is the conjecture that all matter and its interactions can be described as vibrations of unimaginably tiny strings of energy. In simple cases, where distances between objects are large and energy densities are low, string theory would give the same results as general relativity. But it would also explain extreme cases like black holes and the early universe.
Raphael Flauger, a new assistant professor of physics, is trying to piece together a sort of home movie for our universe from back in its fast-growing infancy. The earliest frame we have in this film comes from about 380,000 years after the Big Bang. Taken by satellites, it shows the oldest light in the universe, called the cosmic microwave background (CMB). This ancient glow that filled space has tiny variations in brightness, which suggest that in less than a second after the Big Bang, there were already clumps of energy that would become the seeds of stars and galaxies.
Flauger uses string theory to build models of how the early universe might have evolved.
"You try one version of the film, then see if it fits with the snapshot of the cosmic microwave background," says Flauger. "If it doesn't agree, you throw it out and make another version of the film and see how well it fits."
Flauger is part of a team proposing a new satellite mission that will look for a distinct signature in the cosmic microwave background that could help rule out one or more of the competing models.
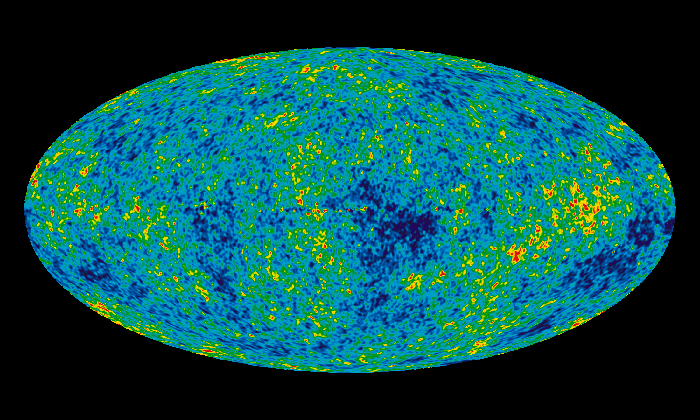
The cosmic microwave background. This detailed, all-sky picture of the infant universe at much less than 1 percent of its current age was produced by the Wilkinson Microwave Anisotropy Probe (WMAP). Credit: NASA / WMAP Science Team
This is the first of a three-part series on general relativity. In part 2 (featuring astronomers Karl Gebhardt and Gary Hill), we explore how the search for dark energy might require a revamp of the equations of gravity. In part 3 (featuring physicist Richard Matzner), we explain how UT scientists have tested the predictions of general relativity for more than four decades.