Bridging Chemistry and Physics on the Path to New Materials
Five questions with Allen J. Bard Center for Electrochemistry director Michael Rose.
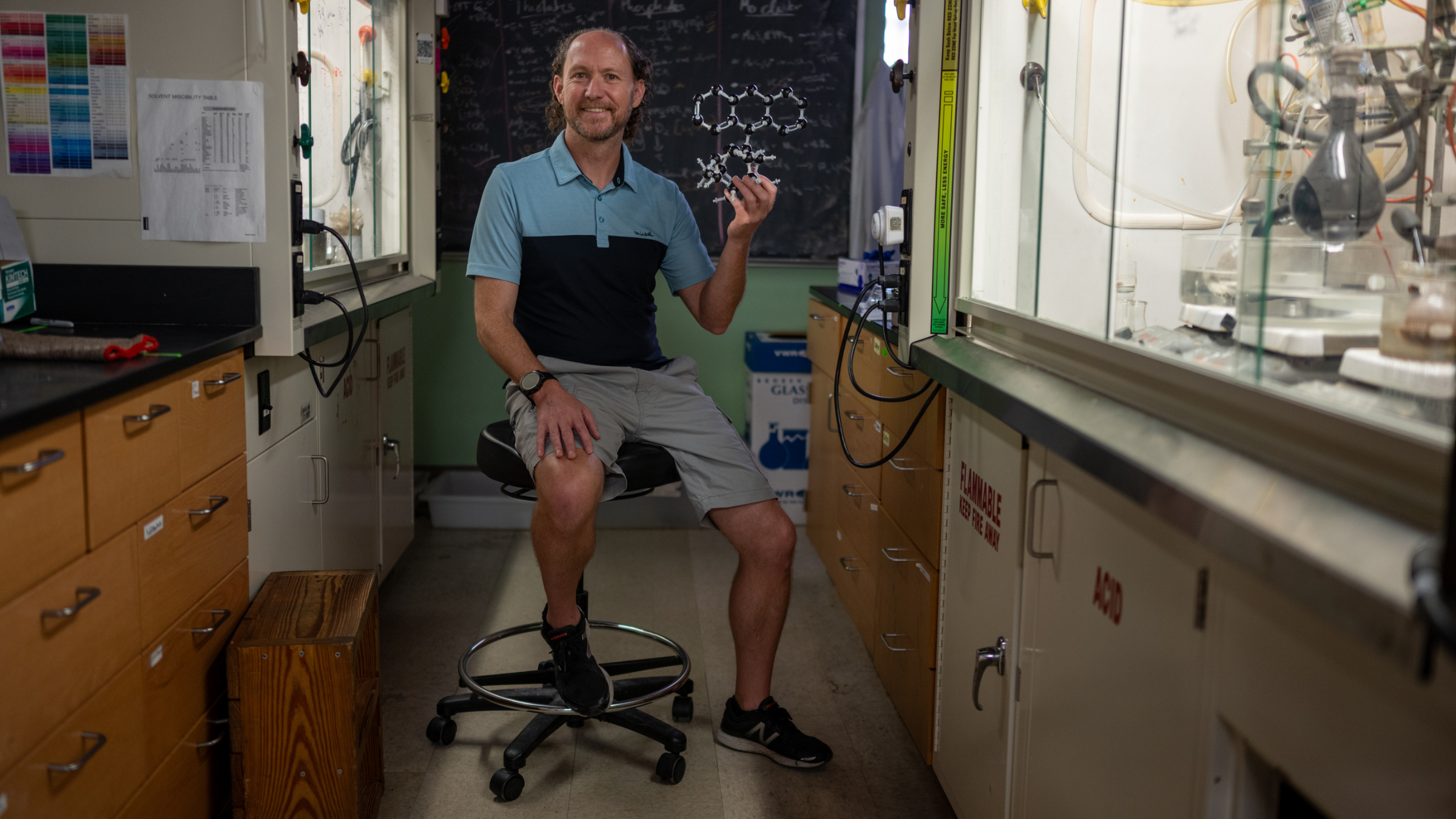
Nolan Zunk
Are there any exciting practical applications for electrochemistry on the horizon or that people might encounter in their everyday lives and might not even realize?
The first application is the ever-miniaturizing domain of semiconductors in all of our computers and iPhones. The size scale of a transistor is so small now that it’s approaching the size of a single molecule. It’s become important to make these transistors out of some combination of material and molecule. My research regarding how molecules can hybridize with materials is very relevant to designing the next generation of transistors.
The other application is the development of new battery materials. Most of our lithium ion batteries are derived from cobalt, which some consider to be a conflict mineral because it’s mined in unsustainable ways. And there’s a drive to make new battery materials out of metals other than cobalt. So what folks are doing now is combining pure metal oxide materials with molecular components that help to improve properties like conductivity and the voltage that you can get out of a battery. That’s another area of electrochemistry where understanding the communication between a molecule part and a material part is really important for driving the new technology and sustainable batteries.
What should people know about your recent research that discussed bridging the gap between chemists’ and physicists’ understanding of solid-state materials?
In the last 10 or 20 years in chemistry, a popular area of research is nanoscience and nanomaterials, which involves the interplay of solid materials with molecules. Molecules are what chemists are good at studying, and solid state materials are what physicists are good at studying. The problem is, we speak different languages about how to make bonds and describe electrons in these two systems.
What my recent research [published this spring in the Journal of the American Chemical Society] has done is try to translate some of the nomenclature from physics into the language of chemistry, so that when chemists talk about making and breaking bonds, we can draw out simple molecular orbital diagrams that help us to understand what type of function we can expect out of materials that are decorated with different sizes and shapes of molecules.
I understand the opportunity to work on this research came about after all of the interruptions to research that were brought about by COVID-19. Were there any special challenges you faced or unexpected opportunities you gained when restarting your research after the pandemic?
There was a delayed effect on my research outcomes and student progress because the students who started during COVID didn’t have as good of access to the lab. That was a challenge that we tried to face together. If there was a silver lining of the pandemic, it was that we learned two or three new computational methods and approaches that we otherwise would probably never have pursued. In the end, we ended up banking these skills and now we use these types of calculations in every paper that we write.
What is the most enjoyable part of leading the Center for Electrochemistry?
The best part about leading the center is that I get to be kept abreast of all of the amazing research that happens in professors’ labs that are associated with the center. Overall, the main goal of the center and the fun part for me is trying to find ways that disparate areas of research and researchers can find common threads to make new collaborations to try to drive new science at the interface between electrochemistry, chemistry and material science.
Are there any misconceptions that you believe the public has about your area of study?
Chemistry sometimes gets bad press because the news headlines are generally focused on bad things that happen. What goes under the radar are all the tremendous benefits that chemistry brings to society, from energy to anti-cancer drugs to insulin sensor systems that improve the lives of people every day.
A misconception about electrochemistry is that it only involves batteries, and in fact, electrochemistry is all around us. Electrochemistry is the way that we see vivid colors on our iPhones. Electrochemistry is how you monitor an EKG. The misconception in electrochemistry is that it’s a narrow field that only does a few things, but it underpins almost every aspect of our economy and health system in one way or another.