The Next 50 Years: A Model of Life on the Atomic Scale
Can we simulate life — in all its messy complexity and at the scale of each individual atom — in a computer?
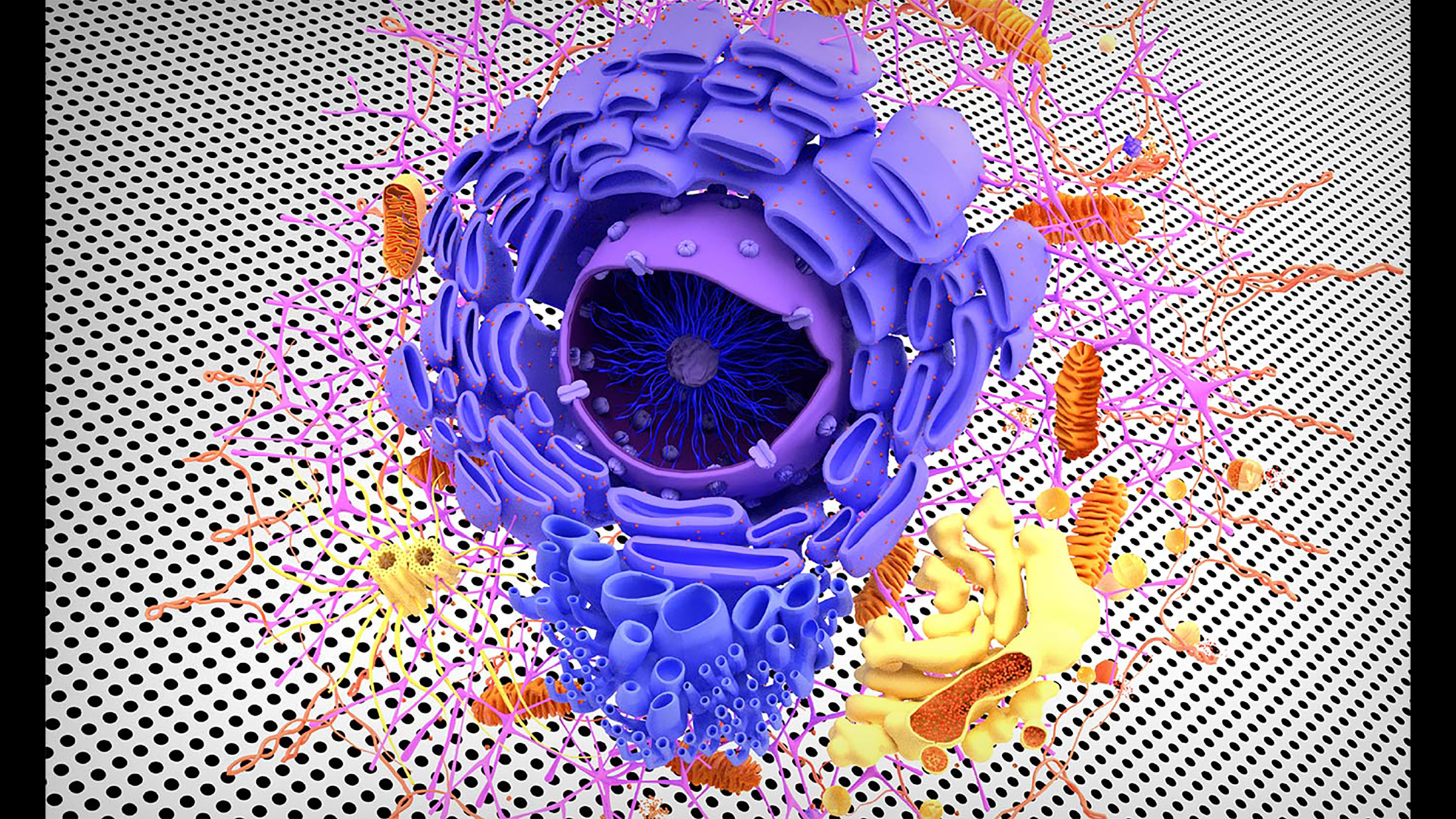
Even the most powerful supercomputers today can only simulate a tiny portion of a single living cell for a few nanoseconds. Carlos Baiz is a biochemist at the University of Texas at Austin who says it might someday be possible to simulate an entire living cell for hours or longer. But he says there are two big catches. Baiz shares his vision for the future in this latest episode of our miniseries, The Next 50 Years.
Show Notes
Music for today's show was produced by:
Podington Bear - https://www.podingtonbear.com/
Chuzausen - https://freemusicarchive.org/music/Chuzausen
TRANSCRIPT
Marc Airhart: This is Marc Airhart for Point of Discovery. We're continuing our series that explores the visions that scientists have for our future, called The Next 50 Years. Can you introduce yourself?
Carlos Baiz: Yeah, my name is Carlos Baiz. I'm an Assistant Professor of Chemistry here at UT. I've been here since 2015.
MA: Baiz told me about this kind of far-out idea that biochemists and computer scientists have been kicking around for a while now …
CB: The vision would be, can we simulate life on a computer from first principles, from physics, basically?
MA: He's talking about a computer model that would simulate the life of just one living cell in the human body. That might resolve some very big unanswered questions at the intersection of many fields of science. It could tell us about how otherwise lifeless matter, chemical molecules, precisely fit together into an intricate and mind-boggling system called life—complex life. The imagined model would account for every single atom in that human cell.
CB: So you would be able to have a movie so to speak, an atomistic movie of how each atom is interacting with each other atom as the cell grows and reproduces. Basically, fully atomistic view of life, which would be an amazing, you know, it's a holy grail, in my opinion. If there's a certain life process that you don't understand, you can go back to the movie right? You'd rewind, play it again, and then see how the molecules interact together and be able to piece together all the different parts.
MA: This kind of simulation might have all kinds of useful applications. You could see in precise detail how cancer or other disorders work. It could reveal why our bodies need sleep, or help us design better drugs. But even better, said Baiz, is the chance to truly understand life at its most basic level.
CB: And you know in 50 years, I don't know what the challenges are going to be. If we think, you know, 50 years in the past. How much did we know about biology? Not … a whole lot. We knew some things, but not a whole lot. So the challenges of today, being able to design drugs and so on, didn't exist 50 years ago, right? So thinking in the future 50 years, I don't really know what the challenges are going to be.
MA: At first, building such a model doesn't sound all that hard. I mean one cell is pretty small. And we already have pretty sophisticated computer models that can tell you what the rain chance is going to be a few days from now across the entire country or model the airflow over the wing of a jet airplane. How hard can modeling one tiny cell be? It turns out there are two big challenges. First, it's going to take A LOT of computer power.
CB: Currently, the best models can simulate a system of a size of about 100 nanometers cube, which is the largest cube in quotes a cube you'll be able to fit inside a cell without running into any of the organelles. So and the timescale for that simulation is about 20 nanoseconds, which is very short on the timescale of life.
MA: He's talking about a model of a small part of a bacterium built by a team from Michigan State University and the RIKEN Advanced Institute for Computational Science in Japan. If you think of a cell as a city, they intentionally picked a quiet neighborhood on the edge of town, rather than the bustling downtown. And they modeled it over a ludicrously short time, like 2 to 2:15 on a Thursday afternoon. So, not really good enough to get a handle on what's really going on in the city. And yet, that required one of the world's most powerful supercomputers.
CB: So now if you say, Well, given that we know how much computer power is needed to simulate all the proteins, right, all the … things that are inside of the cell within this cube, and now we can scale it by the cell volume, right? And then we can estimate a timescale for life, right? How long does it take for a cell to grow and divide? You'd say minutes to hours is kind of the shortest, on the shorter end of the time scale. So based on that, and then following Moore's Law, computational power extrapolating that out to 50 years, it should roughly become possible to simulate an entire cell over a period of minutes in a computer.
MA: So we're going to have to wait a long time for computers to get powerful enough to run a useful simulation of an entire living cell for long enough to see it do interesting things. But scientists like Baiz aren't just sitting around waiting. He's helping solve the other big challenge.
MA: The second big challenge that needs to be overcome to make a true atomistic model of a cell is there's just so much we don't know yet about the basic physics of atoms and molecules that make up cells.
CB: When we talk about, you know, the interior of a cell there are not a whole lot of experiments that tell us how molecules interact with each other inside of a cell. So we need to still work on putting together the single pieces and making sure that those are accurate, and then go into the kind of the full system, would be the other ultimate goal.
MA: Baiz said that much of what we know about how the molecules of living things behave comes from studies where you take a batch of molecules that are all the same and mix a small amount of them into a lot of a solvent, like water. So the molecules aren't bumping into each other much. And there are only two things in the solution, so it's pretty simple. But that's not at all like the real world.
CB: You know, most systems are not just one molecule in one solvent, right? Biology is very complicated. There's lots of different things, lots of different environments. So we're trying to bring this technique into this new era, where we understand the behavior of molecules in environments that resemble biological environments. They're messy, heterogeneous, they have lots of molecules that may or may not be similar to the molecule you're trying to study.
MA: There's not a lot of elbow room inside a cell. Molecules are crowded together like kids packed into a swimming pool on a hot day. Some molecules can move around more easily than others.
CB: Because if you have something that's very small, it can fit through the kind of quote unquote holes and nooks and crannies in the cell. So it can diffuse faster. [If] we have something that's big, now it has to bump into other molecules. So think of it like kind of rocks and sand, right? And water, right? If you have a water molecule, it can fit between the grains of sand. If you have a grain of sand, it can fit between the rocks. But if you have a rock, it can't just push its way through the rest of the solution. A lot of the models are developed from [the] kind of simple perspective of a solution of two different things like a molecule in a solvent, those break down in biological environments. And what my lab does is, we are investigating, how does the environment inside of the cell differ from the kind of liquid dilute solution environment that's relatively well understood at this point.
MA: If Baiz and other biochemists around the world can get a handle on all the physics going on inside messy cells, and if computers can continue to steadily grow in power for 50 years — a believable atom-by-atom model of life itself could be the end result. But either way, the journey might be just as important as the destination.
CB: This is kind of a crazy idea. And I realize it may not be possible, but it's something, it's a very nice concrete, almost like a moonshot-type goal to shoot for, you know, in the next 50 years. Nowadays research is, it's driven by kind of the shorter-term goals, right? Even though those are important. I think that the importance of more applied research is maybe even higher than the fundamental research. But there's a beauty in doing fundamental science for the sake of knowledge, not with any specific goal in mind.
MA: Hey, listeners. Like many of you, I'm hunkered down at home with my family, trying to help flatten the curve of the coronavirus pandemic. I know this is a hard time for many people. I hope you're all being safe and finding ways to stay in touch with your friends and family. We're going to get through this.
MA: Point of Discovery is a production of the University of Texas at Austin's College of Natural Sciences. Music for today's show is by Chuzausen and by Podington Bear. To read a transcript of this show — or find links to more podcasts and essays in The Next 50 Years series — visit us on our website at pointofdiscovery.org. If you like what you heard, be sure and tell your friends. We're available wherever you get your podcasts, including Apple Podcasts, Stitcher and Spotify. Our senior producer is Christine Sinatra. I'm your host and producer Marc Airhart. See you in the future!